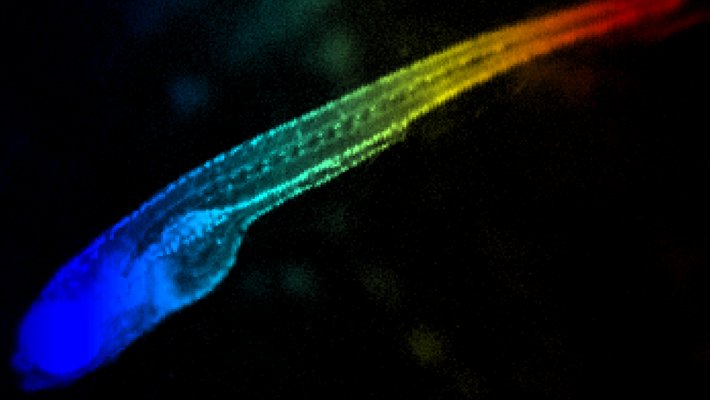
Quantum imaging is a growing field that takes advantage of the counterintuitive and “spooky” ability of light particles, or photons, to become linked, or entangled, under specialized circumstances. If the state of one photon in the entangled duo gets tweaked, so does the other, regardless of how far apart the two photons might be.
how such entanglement could double the resolution of classical light microscopes while also preventing an imaging system’s light from damaging fragile biological samples. Now the same team has improved upon the technique, making it possible to quantum image whole organ slices and even small organisms.
Led by Lihong Wang, the Bren Professor of Medical Engineering and Electrical Engineering, the new work uses entanglement—what Albert Einstein once famously described as “spooky action at a distance”— to control not only the color and brightness of the light hitting a sample, but also the polarization of that light.
“Our new technique has the potential to pave the way for quantum imaging in many different fields, including biomedical imaging and potentially even remote space sensing,” says Wang, who is also the Andrew and Peggy Cherng Medical Engineering Leadership Chair and executive officer for medical engineering.
Like wavelength and intensity, polarization is a fundamental property of light and represents which direction the electric component of a light wave is oriented with respect to the wave’s general direction of travel. Most light, including sunlight, is unpolarized, meaning that its electromagnetic waves move and travel in all directions. However, filters called polarizers can be used to create light beams with one specific polarization. A vertical polarizer, for example, only allows photons with vertical polarization to pass through. Those with horizontal polarization (meaning that the electric component of the light wave is oriented horizontally with respect to the direction of travel) will be blocked. Any light with other polarization angles (between vertical and horizontal), will partially pass through. The outcome is a stream of vertically polarized light.
This is how polarized sunglasses reduce glare. They use a vertically polarizing chemical coating to block sunlight that has become horizontally polarized by reflecting off a horizontal surface, such as a lake or snowy field. This means that the wearer only observes vertically polarized light.
When changes in light intensity or color are not enough to give scientists quality images of certain objects, controlling the polarization of the light in an imaging system can sometimes provide more information about the sample and offer a different way to identify contrast between a sample and its background. Detecting the changes in polarization caused by certain samples can also give researchers information about the internal structure and behavior of those materials.
Wang’s newest microscopy technique, dubbed quantum imaging by coincidence from entanglement (ICE), takes advantage of entangled photon pairs to obtain higher-resolution images of biological materials, including thicker samples, and to make measurements of materials that have what scientists call birefringent properties.
Rather than consistently bending incoming light waves in the same way, as most materials do, birefringent materials bend those waves to different degrees depending on the light’s polarization and the direction in which it is traveling. The most common birefringent materials studied by scientists are calcite crystals. But biological materials, such as cellulose, starch, and many types of animal tissue, including collagen and cartilage, are also birefringent.
If a sample with birefringent properties is placed between two polarizers oriented at 90-degree angles to each other, some of the light going through the sample will be altered in its polarization and will therefore make it through to the detector, even though all the other incoming light should be blocked by the two polarizers. The detected light can then provide information about the structure of the sample. In materials science, for example, scientists use birefringence measurements to get a better understanding of the areas where mechanical stress builds up in plastics.
In Wang’s ICE setup, light is passed first through a polarizer and then through a pair of special barium borate crystals, which will occasionally create an entangled photon pair; about one pair is produced for every million photons that pass through the crystals. From there, the two entangled photons will branch off and follow one of the system’s two arms: one will travel straight ahead, following what is called the idler arm, while the other traces a more circuitous path called the signal arm that causes the photon to pass through the object of interest. Finally, both photons go through an additional polarizer before reaching two detectors, which record the time of arrival of the detected photons. Here, though, occurs a “spooky” quantum effect because of the entangled nature of the photons: the detector in the idler arm can act as a virtual “pinhole” and “polarization selector” on the signal arm, instantly affecting the location and polarization of the photon incident on the object in the signal arm.
“In the ICE setup, the detectors in the signal and idler arms function as ‘real’ and ‘virtual’ pinholes, respectively,” says Yide Zhang, lead author of the new paper and a postdoctoral scholar fellowship trainee in medical engineering at Caltech. “This dual pinhole configuration enhances the spatial resolution of the object imaged in the signal arm. Consequently, ICE achieves higher spatial resolution than conventional imaging that utilizes a single pinhole in the signal arm.”
“Since each entangled photon pair always arrives at the detectors at the same time, we can suppress noises in the image caused by random photons,” adds Xin Tong, co-author of the study and a graduate student in medical and electrical engineering at Caltech.
To determine the birefringent properties of a material with a classical microscopy setup, scientists typically switch through different input states, illuminating an object separately with horizontally, vertically, and diagonally polarized light, and then measuring the corresponding output states with a detector. The goal is to measure how the birefringence of the sample alters the image that the detector receives in each of those states. This information informs scientists about the structure of the sample and can provide images that would not otherwise be possible.
Since quantum entanglement allows paired photons to be linked no matter how far apart they might be, Wang is already imagining how his new system could be used to make birefringence measurements in space. Consider a situation where something of interest, perhaps an interstellar medium, is located light years away from Earth. A satellite in space might be positioned such that it could emit entangled photon pairs using the ICE technique, with two ground stations acting as detectors. The large distance to the satellite would make it impractical to send any kind of signal to adjust the device’s source polarization. However, due to entanglement, changing the polarization state in the idler arm would be equivalent to changing the polarization of the source light before the beam hits the object. “Using quantum technology, nearly instantaneously, we can make changes to the polarization state of the photons no matter where they are,” Wang says. “Quantum technologies are the future. Out of scientific curiosity, we need to explore this direction.”
, “Quantum imaging of biological organisms through spatial and polarization entanglement,” appears in the March 8 issue of the journal Science Advances. In addition to Wang, Zhang, and Tong, the paper’s co-authors are medical engineering graduate student David Garrett, postdoctoral scholar research associate Rui Cao, and former postdoctoral scholar research associate Zhe He, who is now at the Shandong Institute of Advanced Technology. The work was supported by funding from Caltech’s Center for Sensing to Intelligence and the National Institutes of Health.